Cancer
Cancer cells rapidly take in large amounts of nutrients in order to maintain active cell growth. They metabolize these nutrients to synthesize and nucleic acids and to produce energy such as ATP. Even under unfavorable conditions, such as hypoxia or low nutrition, cancer cells can survive by altering their metabolic systems. Therefore, the metabolic systems of cancer cells have attracted many researchers’ attention.
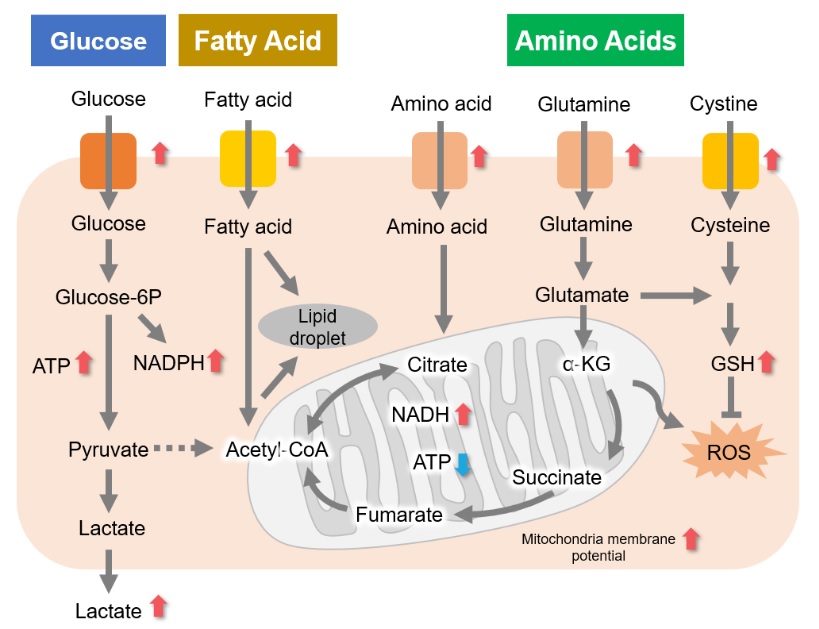
A recurring characteristic of cancer cell metabolism is that cancer cells generally prefer to produce ATP via the glycolytic system despite that system being less efficient than mitochondrial oxidative phosphorylation (Warburg effect). As a result, cancer cells take up large amounts of glucose. They also produce a large amount of lactate due to increased glycolytic activity. This method of ATP production allows cancer cells to proliferate even under hypoxia, because the glycolytic system does not require oxygen. Meanwhile, the mitochondria of cancer cells use amino acids and fats to produce NADH. It is commonly recognized that NADH in cancer cell mitochondria is mainly used for redox regulation in addition to ATP production. The abnormal functions of mitochondria in cancer cells result in increased mitochondrial membrane potential (hyperpolarization) and excessive ROS production. Consequently, they produce large amounts of glutathione to maintain the redox balance. Since glutamine and cysteine are essential nutrients for glutathione production, cancer cells take up large amounts of these amino acids. Additionally, since NADPH is required to maintain the reduction of glutathione, the pentose phosphate pathway (downstream from the glycolytic system) and NADH in mitochondria are used to maintain high NADPH levels.
(Note) The above information represents the general metabolic characteristics of cancer cells and may vary depending on the type of cancer cell and its environment.
References:
Below articles are general review on cancer cell metabolism.
1) Glycolytic system:
M. G. Vander Heiden, L. C. Cantley, and C. B. Thompson, “Understanding the Warburg Effect: The Metabolic Requirements of Cell Proliferation”, Science, 2009, 324, 1029.
2) Amino Acids metabolism, ROS:
P. Koppula, Y. Zhang, and B. Gan, “Amino Acid Transporter SLC7A11/xCT at the Crossroads of Regulating Redox Homeostasis and Nutrient Dependency of Cancer”, Cancer Commun., 2018, 38, 12.
3) Amino Acids:
E. L. Lieu, T. Nguyen, S. Rhyne, and J. Kim, “Amino Acids in Cancer”, Exp. Mol. Med., 2020, 52, 15.
4) Mitochondrial, ROS, NADPH:
F. Ciccarese and V. Ciminale, “Escaping Death: Mitochondrial Redox Homeostasis in Cancer Cells”, Front. Oncol., 2017, 7, 117.
5) NADH:
A. Chiarugi, C. Dolle, R. Felici, and M. Ziegler, “The NAD Metabolome-A Key Determinant of Cancer Cell Biology”, Nat. Rev. Cancer, 2012, 12, 741.
Cellular Metabolism Related Disease
- Glucose Metabolism Inhibition and Anticancer Effects
- Amino Acid Metabolism Inhibition and Anticancer Effects
- Fatty Acid Metabolism Inhibition and Anticancer Effects
- Cancer Immunity and Metabolism
Glucose Metabolism Inhibition and Anticancer Effects
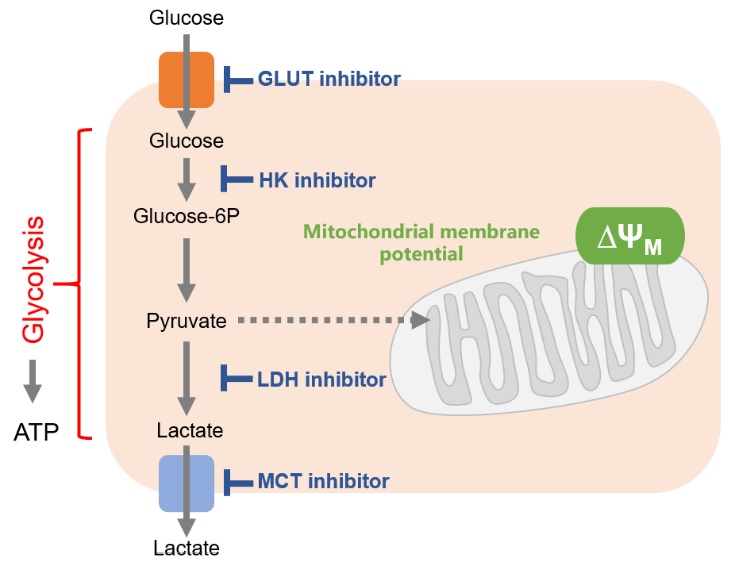
Cancer cells mainly use the glycolytic system to produce ATP. Thus, the glycolytic system is the most important pathway to understand in the metabolism of cancer cells. Consequently, the glycolytic system has long been a target of anticancer drug development. Although effective anticancer drugs have not yet been developed, the glycolytic system remains a major drug target.
One of the drug discovery target proteins of the glycolytic system is the glucose transporter (GLUT). Since cancer cells take up large amounts of sugar via glucose transporters, it is possible to suppress the glycolytic system by directly inhibiting glucose transporters. Inhibition of the enzymes responsible for glucose starvation and glycolysis [hexokinase (HK), lactate dehydrogenase (LDH), etc] as well as inhibition of the efflux of lactate (the end product of glycolysis) are also effective.
Changes in Intracellular Metabolism by Each Inhibitor / References
Cell Line |
Inhibitor / Inducer |
Changes to Cellular Metabolism |
Publication |
SKOV3, OVCAR3, HEY, A2780
|
GLUT1 inhibitor: BAY-876
|
ATP ↓, Lactate ↓
|
Cancer, 2019, 11, 33
|
H1299, H460, H2030
|
GLUT1 express inhibitor: Apigenin |
Glucose consumption ↓
Lactate ↓, ATP ↓
NADPH ↓, GSH/GSSG ↓
ROS ↓
|
Int. J. Oncol., 2016, 48, 399
|
HCT116
|
HK inhibitor: 2-DG |
Glucose uptake ↓
Lactate ↓, Acetyl-CoA ↓
H3K27Ac ↓
|
Cancer Metab., 2015, 3, 10
|
SKOV3, HEY
|
HK inhibitor: 2-DG + Metformin |
ATP ↓, Lactate ↓
|
Am. J. Transl. Res., 2016, 8, 4821
|
CT26
|
Complex I inhibitor: Phenformin,
LDH inhibitor: Oxamate
|
Glucose uptake ↓
Lactate ↓, ATP ↓
ROS ↑
|
PLoS One., 2014, 9, e85576
|
P493
|
LDH inhibitor: FX11 |
ATP ↓, Lactate ↓
NADH/NAD ↑, ROS ↑
Mitochondrial MP ↓
|
Proc. Natl. Acad. Sci. USA, 2010, 107(5), 2037
|
Raji
|
MCT inhibitor: AZD3965 |
Glucose uptake ↓
Intracellular Lactate ↑
(Extracellular Lactate ↓)
|
Cancer Res., 2017, 77(21), 5913
|
Related Products
Amino Acid Metabolism Inhibition and Anticancer Effects
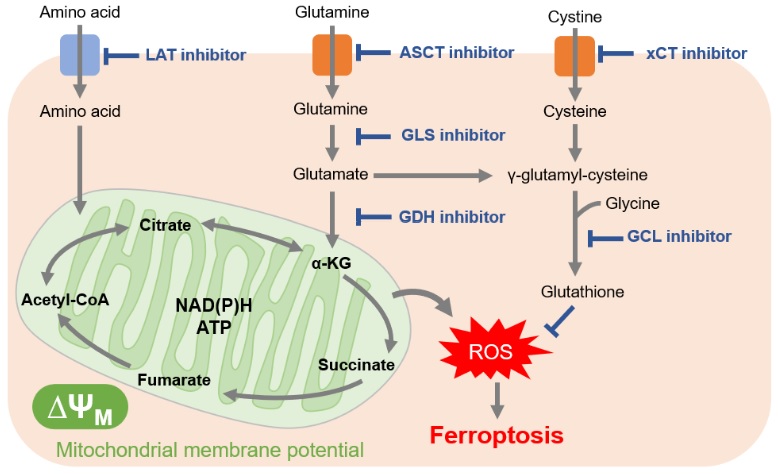
In cancer cells, which are actively proliferating, amino acids are essential for the synthesis of proteins and nucleic acids. Furthermore, many cancer cells downregulate acetyl CoA production from pyruvate, requiring cancer cells to also use amino acids as nutrient sources for the TCA cycle. This explains why cancer cells have been shown to increase expression of amino acid transporters to take up large amounts of amino acids.
Glutamine in particular is a raw material for glutathione and a source of α-ketoglutarate, which is essential for the TCA cycle. For these reasons, glutamine uptake and glutaminolysis (glutamine metabolism) have attracted attention as drug targets. As well, the amino acid transporter LAT (L-type amino acid transporter), which is involved in the uptake of many essential amino acids, was found to be overexpressed in many cancer cells. LAT is expected to a be future drug target.
Because cancer cells produce a large amount of reactive oxygen species, they maintain redox balance by increasing the production of glutathione, an antioxidant. Thus, inhibition of the pathways involved in glutathione production can change the intracellular redox balance and induce methods of cell death such as ferroptosis. In addition to the reason just stated, glutathione also contributes to drug resistance, which is why the pathway involved in glutathione production has become a major target for drug development.
Cysteine is an amino acid also required for redox regulation and is mainly taken up into cells by the cystine transporter (xCT). Sulfasalazine, long used as an anti-inflammatory drug, and sorafenib, a molecular targeted therapy for cancer, have recently been shown to inhibit xCT. For the same cell death-mediated anticancer effect as glutathione inhibition, xCT has also attracted attention as a target for drug development.
Changes in Intracellular Metabolism by Each Inhibitor / References
Cell Line |
Inhibitor / Inducer |
Changes to Cellular Metablism |
Publication |
KO99L
|
LAT1 inhibitor: JPH203
|
Mitochondrial MP ↓
Autophagy ↑
|
Leukemia, 2015, 29, 1253
|
LS174T
A549
|
LAT1 inhibitor: JPH203 |
Glutamine uptake ↓
Leucine uptake ↓
|
J. Biol. Chem., 2018, 293(8), 2877
|
HeLa
|
LAT1 inhibitor: BCH |
Tryptophan uptake ↓
|
J. Immunol., 2011, 187(4), 1617
|
A549
|
ASCT2 inhibitor: GPNA |
Glutamine uptake ↓
ROS ↑
|
Clin. Cancer Res., 2013, 19(3), 560
|
MG63.3
|
GLS inhibitor: CB839 |
Glutamine ↑
Glutamate ↓, GSH ↓
|
Cancer&Metab., 2020, 8:4
|
OCI-AML3
|
GLS inhibitor: CB839 |
ATP ↓, NADH/NAD ↓
GSH/GSSG ↓, Alanine ↓
Glutamate ↓
|
Mol. Cancer Ther., 2019, 18(11), 1937
|
H1299
MDA-MB231
|
GDH inhibitor: R162 |
ROS ↑
GPx activity ↓
|
Cancer Cell, 2015, 27, 257
|
A2780
|
xCT inhibitor: Erastin |
Cystine uptake ↓
GSH ↓
|
Sci. Rep., 2018, 8(1), 968
|
B16-F10
|
xCT inhibitor: Sulfasalazine |
GSH ↓
ROS ↑
|
PLoS One., 2018, 13(4), e0195151.
|
HT-1080
|
xCT inhibitor: Sorafenib |
Cystine uptake ↓, GSH ↓
Lipid peroxidation ↑
|
Elife, 2014, 3, e02523
|
PANC-1
|
GCL inhibitor: BSO |
GSH ↓
Lipid peroxidation ↑
|
Oncol. Lett., 2018, 15(6), 8735
|
A549
|
GCL inhibitor: BSO
|
Cystine uptake ↓, GSH ↓
|
Toxicol. Appl. Pharmacol., 1985, 381
|
Related Products
Fatty Acid Metabolism Inhibition and Anticancer Effects
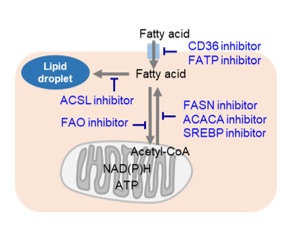
Cancer cells, which are actively proliferating, naturally require a large amount of lipids. Thus, intracellular fatty acid synthesis and extracellular fatty acid uptake are both very active. Therefore, many cancer cells have enhanced lipid droplet accumulation. Consequently, the pathway involved in fatty acid production is a very popular therapeutic target for cancer, and many inhibitors have been developed. Additionally, cancer cells perform β-oxidation of fatty acids for efficient energy production in order to compensate for inefficient energy production by the glycolytic system. Thus, drugs targeting the β-oxidation of fatty acids are also being developed.
Changes in Intracellular Metabolism by Each Inhibitor / References
Cell Line |
Inhibitor / Inducer |
Changes to Cellular Metabolism |
Publication |
CD36 Highly expressed MCF7, SUM159
|
CD36 inhibitor: SSO
|
Fatty acid uptake ↓
Lipid droplet ↓
|
Cancers, 2019, 11, 2012
|
A375, SKMel28
|
FATP inhibitor: Lipofermata |
Fatty acid uptake ↓
Lipid droplet ↓
|
Cancer Discov., 2018, 8(8), 1006
|
BT474, MCF7, T47D
|
ACACA inhibitor: TOFA |
Lipid droplet ↓
FA oxidation ↓
|
J. Clin. Med., 2020, 9, 87
|
ccRCC
|
SREBP inhibitor: Betulin
ACACA inhibitor: TOFA
FASN inhibitor: C75
|
Lipid droplet ↓
|
Mol. Cell Biol., 2017, 37(22), e00265-17
|
NCI-H1703
|
ACSL inhibitor: Triacsin C |
Lipid droplet ↓
|
Int. J. Cancer, 2020, 147, 1680
|
UM-UC-3
|
FAO inhibitor: Etomoxir |
Lipid droplet ↑
ATP ↓, NADPH ↓
|
Clin. Sci., 2019, 133 (15), 1745
|
SF188
|
FAO inhibitor: Etomoxir |
ATP ↓, NADPH ↓
GSH ↓
ROS ↑
|
Biochim. Biophys. Acta, 2011, 1807(6), 726
|
Related Products
Cancer Immunity and Cellular Metabolism
T cells play a central role in the immune system by eliminating cancer cells. In recent years, it has become clear that metabolism is also involved in the regulation of T cell functions such as differentiation and activation, inspiring more active research on metabolism in cancer immunity.
Cancer cells take in large amounts of nutrients to maintain their proliferative activity. Activated T cells also require large amounts of nutrients to eliminate cancer cells. Cancer cells and activated T cells thus compete for nutrients – particularly glucose.
Activated T cells express the PD-1 immune checkpoint receptor on their surface. Cancer cells, in turn, express PD-L1, as the interaction will suppress T cell glucose uptake. It is in this way that cancer cells will regulate the metabolism of immune cells in order to evade the immune system. Therefore, it is important to understand the metabolism of not only cancer cells but also immune cells for the sake of cancer immunology.
References:
1) Z. Yin, L. Bai, W. Li, T. Zheng, H. Tian, and J. Cui, “Targeting T cell metabolism in the tumor microenvironment: an anti-cancer therapeutic stratety”, J. Exp. Clin. Cancer Res. 2019, 38, 403.
2) L. Almeida, M. Lochner, L. Berod, and T. Sparwasser, “Metabolic pathways in T cell activation and linear differentiation”, Semin. Immunol. 2016, 28(5), 514.
3) A. Kumar and K. Chamoto, “Immune metabolism in PD-1 blockage-based cancer immunotherapy”, Int. Immunol., 2020 Jul 5;dxaa046.
4) D. G. Franchina, F. He, and D. Brenner, “Survival of the fittest: Cancer challenges T cell metabolism”, Cancer Lett., 2018, 412, 216.
5) N. Patsoukis, K. Bardhan, P. Chatterjee, D. Sari, B. Liu, L. N. Bell, E. D. Karoly, G. J. Freeman, V. Petkova, P. Seth, L. Li, and V. A. Boussiotis, “PD-1 alters T-cell metabolic reprogramming by inhibiting glycolysis and promoting lipolysis and fatty acid oxidation”, Nat. Commun., 2015, 6, 6692.
Changes in Intracellular Metabolism by Each Inhibitor / References
Cell Line
|
Antigen Activation
|
Changes to Cellular Metabolism
|
Publication
|
T cell
|
Activation
|
Glucose uptake ↑
|
J. Immunol., 2008, 180, 4476
|
CD8(+) T cell
|
Activation
|
Glucose uptake ↑
FAO ↓
|
J. Clin. Invest., 2013, 123, 4479
|
CD4(+) T cell
|
Activation
|
Glucose uptake ↑
Lactate ↑, ATP ↑
|
eLife, 2018, 7, e30938
|
CD4(+) T cell
|
Activation
|
Glucose uptake ↑, Lactate ↑
Glutamine uptake ↑
FAO ↓
|
Nat. Commun., 2015, 6, 6692 |
CD4(+) T cell |
Activation
(Interactions between PD-1 & PDL1)
|
Glucose uptake ↓, Lactate ↓
Glutamine uptake ↓
FAO ↑
|
Related Products
Senescence
- Relationship between Senescence-Related Diseases and Lactate/NAD+
- Senescence due to DNA Damage
- Glutamine Metabolism and Cellular Senescence
Relationship Between Senescence-Related Diseases and Lactate/NAD+
Recently, the relationship between NAD+ and senescence has been attracting attention. In the mouse aging model, a decreased amount of NAD+ was observed in the liver and other organs,1 whereas inhibition of NAD+ synthase has been reported to induce senescence-like decline in various cellular functions.2 Additionally, it has been suggested that a decrease in in NAD+ levels leads to decreased mitochondrial function,3 whereas a decrease in mitochondrial function has similarly been suggested to lead to a decrease in NAD+ levels and a senescence-like decline in cellular function.4
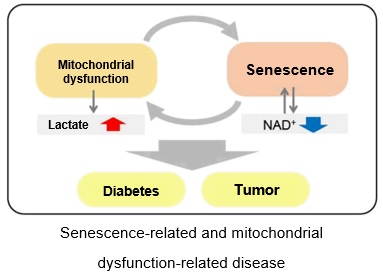
DNA Damage-Triggered Senescence
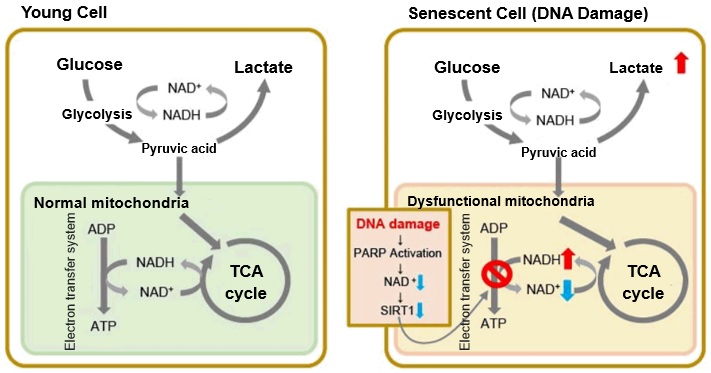
When mitochondrial function declines, ATP becomes more predominantly produced via anaerobic glycolysis, resulting in increased lactate production. DNA damage is one way cellular senescence can cause mitochondrial dysfunction.
The accumulation of DNA damage activates DNA repair mechanisms and increases NAD+ consumption. The decrease in available NAD+ decreases the activity of SIRT1, an important factor in maintaining mitochondrial function. Mitochondrial dysfunction results as decreased SIRT1 activity leads to inhibition of electron transfer, resulting in reduced ATP production and NAD+.3,8
Glutamine Metabolism and Cellular Senescence
Tumor suppressor menin prevents effector CD8 T cell dysfunction by targeting mTORC1-dependent metabolic activation9
Menin is a tumor suppressor that plays an important role preventing T cell dysfunction from aging and fatigue. When menin is deficient, mTORC1 is activated, and oxidative phosphorylation via glycolysis and glutaminolysis is enhanced, leading to CD8 T cell dysfunction. Additionally, α-ketoglutarate, an intermediate product of glutamine metabolism, maintains the activation of mTORC1 and contributes to the promotion of cellular senescence (increased SA-β-gal activity). It has been suggested that the glutamine-α ketoglutarate pathway plays an important role in the induction of CD8 T cell dysfunction and that menin may inhibit T cell senescence.
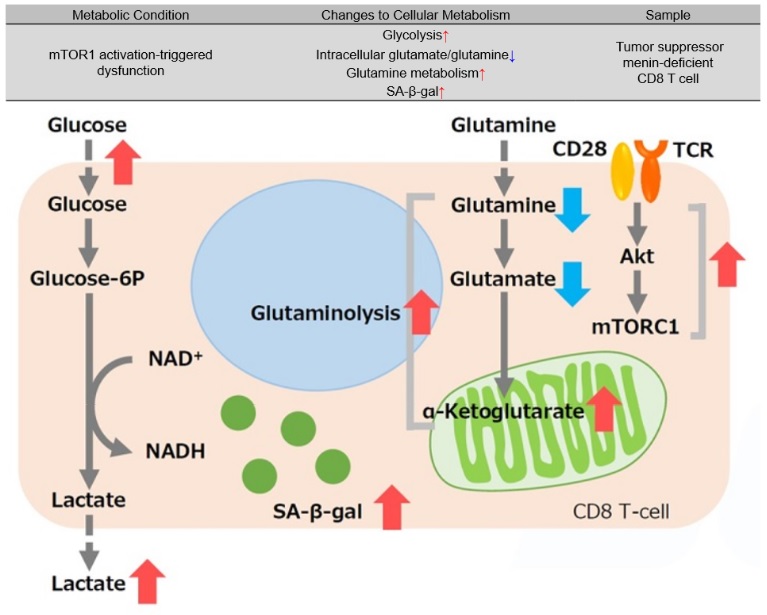
References:
1) J. Yoshino, K. F. Mills, M. J. Yoon and S. Imai, “Nicotinamide mononucleotide, a key NAD+ intermediate, treats the pathophysiology of diet- and age-induced diabetes in mice”, Cell Metab., 2011, 14(4), 528.
2) L. R. Stein and S. Imai, “Specific ablation of Nampt in adult neural stem cells recapitulates their functional defects during aging”, EMBO J., 2014, 33(12), 1321.
3) J. Wu, Z. Jin, H. Zheng and L. Yan, “Sources and implications of NADH/NAD+ redox imbalance in diabetes and its complications”, Diabetes Metab. Syndr. Obes., 2016, 9, 145.
4) A. Bratic and N. Larsson, “The role of mitochondria in aging”, J Clin Invest. 2013, 123(3), 951.
5) I. San-Millancorresponding and G. A. Brooks, “Reexamining cancer metabolism: lactate production for carcinogenesis could be the purpose and explanation of the Warburg Effect”, Carcinogenesis, 2017, 38(2), 119.
6) Y. Wu, Y. Dong, M. Atefi, Y. Liu, Y. Elshimali, and J. V. Vadgama, “Lactate, a Neglected Factor for Diabetes and Cancer Interaction”, Mediators Inflamm., 2016. DOI: 10.1155/2016/6456018
7) Z. Feng, R. W. Hanson, N. A. Berger and A. Trubitsyn, “Reprogramming of energy metabolism as a driver of aging”, Oncotarget., 2016, 7(13), 15410.
8) S. Imai and L. Guarente, “NAD+ and sirtuins in aging and disease”, Trends in Cell Biology, 2014, 24(8), 464.
9) J. Suzuki, et al, “The tumor suppressor menin prevents effector CD8 T-cell dysfunction by targeting mTORC1-dependent metabolic activation.”, Commun Nat Commun, 2018, 9(1), 3296.
Related Products
NASH
Nonalcoholic steatohepatitis (NASH)
Nonalcoholic fatty liver disease (NAFLD) is a pathological change in the liver that occurs predominantly in the elderly, regardless of drinking history. NAFLD is a comprehensive disease concept that progresses from fatty liver (steatosis) through nonalcoholic steatohepatitis (NASH) to fibrosis/cirrhosis and finally hepatocellular carcinoma (HCC). 1
Recently, it has been reported that cellular senescence and associated mitochondrial dysfunctions contribute significantly to the development of NAFLD/NASH, and these reports have attracted much attention. 2,3,4,5
Obesity, a risk factor for NAFLD/NASH, increases lipid deposition, which then promotes the production of reactive oxygen species (ROS) and inflammatory cytokines, leading to cellular senescence. 2 The decrease in NAD+ levels associated with cellular senescence results in a decrease in mitochondrial function. 3 The decrease in mitochondrial function then leads to a further decrease in NAD+ levels, which exacerbates senescence-like changes in cellular function. 4
On the other hand, liver-specific induction of cellular senescence has been shown to increase lipid deposition and promote fibrosis.5 This indicates that cellular senescence, abnormal mitochondrial function, and associated metabolic changes produce a synergy in the development of NAFLD/NASH, forming inflammatory and fibrotic pathologies.
In the future, more detailed analysis of the relationship between this complex synergy and NAFLD/NASH is expected to contribute to the establishment of new treatments.
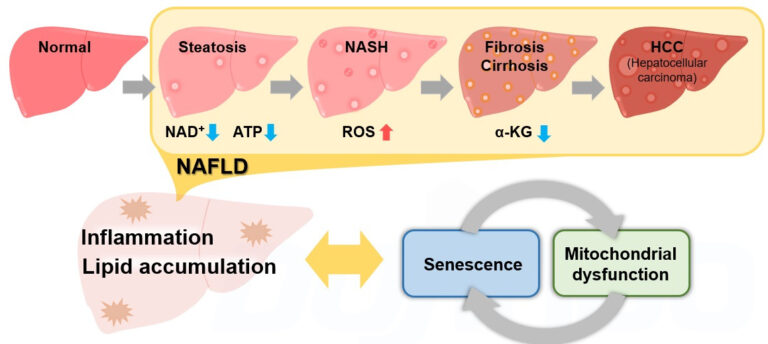
Reported cases in each NAFLD/NASH model
* Click on the links in the table to visit the store pages of these measurement kits/reagents
NAFLD/NASH model |
Cellular functions, metabolic changes, etc. |
References |
Mouse model of aging, obesity, and diabetes
|
Hepatocyte senescence(SA-ß-Gal ↑, p21 ↑)
⇒ Removal of senescent cells improves healthspan
|
Nat. Commun., 2016, 8, 15691.
|
Mice treated with high-fat + high-cholesterol diet
|
Abnormal mitochondrial function in the liver
(ATP ↓,membrane potential ↓,hydrogen peroxide production ↑)
|
Redox Biol., 2018, 15, 86-96
|
Mice treated with high-fat diet
|
Abnormal mitochondrial function in liver(ATP ↓,NAD ↓),PARP ↑
⇒ inhibition of PARP expression improves mitochondrial function and medical condition
|
J. Hepatol., 2017, 66, 132–141
|
Hepatic astrocyte fibrosis model
|
a-ketoglutarate ↑,type I collagen ↑
→ addition of a-ketoglutarate improves fibrosis
|
Biosci. Rep., 2020, 40, BSR20193385
|
High-fat diet treated mice
|
Lipid deposition in liver ↑,liver function ↓
→ addition of a-ketoglutarate improves the condition
|
Liver Res., 2020, 4, 94e10095
|
Reference:
1) T. Hardy, F. Oakley, Q. M. Anstee and C. P. Day, “Nonalcoholic fatty liver disease: pathogenesis and disease spectrum”, Annu. Rev. Pathol., 2016, 11, 451-496.
2) M. J. Schafer, et al, “Exercise Prevents Diet-Induced Cellular Senescence in Adipose Tissue”, Diabetes, 2016, 65, 1606-1615.
3) J. Wu, Z. Jin, H. Zheng and L. Yan, “Sources and implications of NADH/NAD+ redox imbalance in diabetes and its complications”, Diabetes Metab. Syndr. Obes., 2016, 9, 145.
4) A. Bratic and N. Larsson, “The role of mitochondria in aging”, J Clin Invest. 2013, 123(3), 951.
5) M. Ogrodnik, et al, “Cellular senescence drives age-dependent hepatic steatosis”, Nat. Commun., 2016, 8, 15691.
Others
Glutamine Metabolism and Autophagy
Promotion of autophagy and glutaminolysis via stabilization of glutaminase by BAG3
BAG3 is a protein involved in the regulation of various cellular functions, such as apoptosis, cell differentiation, and macroautophagy, etc. In cells overexpressing BAG3, glutaminase (GLS) succinylation is promoted, which prevents ubiquitination and subsequent proteosomal degradation, stabilizing the GLS protein. Stabilization of GLS promotes glutaminolysis, which in turn increases glutamine consumption. This results in increased intracellular glutamate and increased intracellular α-ketoglutarate, an intermediate product of glutamine metabolism. Accelerated glutaminolysis produces ammonia in the culture medium, which contributes to the activation of autophagy. For cancer cells with enhanced glutaminolysis, BAG3 has been suggested as a potential target for cancer therapy.
* BAG3 is a protein that is ubiquitously distributed in human tissues and is induced by various stresses. BAG3 is a protein involved in the regulation of various cellular functions and is also associated with cancer and age-related neurodegenerative diseases.
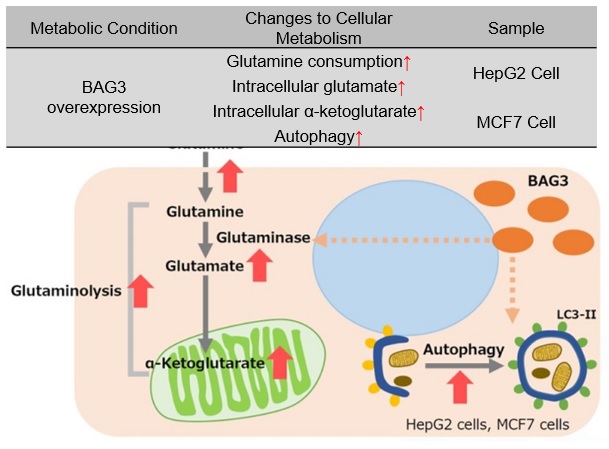
Reference:
S. Zhao, et al, “BAG3 promotes autophagy and glutaminolysis via stabilizing glutaminase”, Cell Death & Disease, 2019, 284(10).
Related Products